Extraction of Capsaicin: Formulation and Evaluation of a Transdermal Patch
Keywords:
Transdermal patches, Capsaicin, Anti-inflammatory, Endurance test, Permeation enhancer, TDDSSynopsis
Ethyl ester prodrug of non-sulfhydryl angiotensin converting enzyme (ACE) inhibitor capsaicinat is the proposed model drug capsaicin. These hypothesized TDDs managed ventricular arrhythmias and had short biological half-life, low oral bioavailability, dosage, and molecular weight for better drug absorption. The suggested transdermal patch combines a slow-release calcium channel blocker with a sustained-release capsaicin angiotensin converting enzyme inhibitor. The prepared capsaicin film was characterized by “optical checking, smoothness color, transparency and flexibility, thickness of polymeric films, mass deviation, uniformity or texture, surface pH, tensile strength, cracking acceptance power, water ingestion amount, swelling ratio, wetness, and in-vitro drug release study”. Hydrophilic polymers chitosan increased water-soluble capsaicin spreadability and regulated drug release by 95.5% in 12 hours. The polymeric films (TLF6) were chosen for their look, tensile strength, percentage elongation, folding endurance, swelling ratio, moisture content, moisture uptake nature, drug content, and in-vitro drug release study parameters. Release kinetics showed that the produced film followed diffusion kinetics and released immediately. The release kinetic investigation showed that the patch followed super case II diffusion kinetics with sustained release over time. After regression analysis, slope values were calculated from the graph and r2 values indicated linearity.
Topical drug delivery system
Easy use and good patient compliance make oral drug administration the most popular. Its drawbacks include hepatic first-pass metabolism requiring greater medication dosages and stomach discomfort from surfactants in lipid-based formulations. Unintended adverse effects are common with systemic drug delivery. Non-invasive, non-painful, and non-irritating topical drug delivery devices may overcome these constraints. These systems use targeted drug delivery to lower systemic toxicity, first-pass metabolism, gastrointestinal irritation, and percutaneous absorption, and boost bioavailability with sustained release characteristics. Despite these benefits, typical transdermal formulations including ointments, creams, and lotions have stickiness, poor spreadability, and stability concerns that cause patient non-compliance. Topical medication distribution is difficult because the stratum corneum is difficult to penetrate. This 40% protein, 40% lipid, and 20% water layer with tight intercellular connections is a stronger barrier than the gastrointestinal, rectal, buccal, nasal, and vaginal membranes. Despite these obstacles, topical medication administration can be used for site-specific or systemic effects when other routes fail. It effectively treats fungal infections. Recent advances in semisolid transparent gel compositions have broadened cosmetic and medicinal applications. These compositions improve stability, spreadability, and aesthetics over standard preparations. Formulation scientists still struggle to create efficient topical dose formulations.
Topical drug delivery systems are unique ways to deliver drugs directly to the skin or mucosal surfaces for localized and systemic effects. This approach is used for skin problems, pain treatment, and cosmetics. Topical drug delivery systems avoid first-pass metabolism and reduce systemic side effects, making them a good alternative to oral or injectable drugs. These devices' ease of use and non-invasiveness improve patient compliance, making them popular in clinical and consumer sectors. Topical drug delivery systems can transport medications directly to the skin's surface or deeper layers, depending on the therapeutic impact. The stratum corneum, the skin's outermost layer, protects it from foreign contaminants. Advanced formulations use penetration enhancers, lipid-based systems, and nanotechnology to bypass this barrier. These technologies allow active medicinal compounds to infiltrate the skin and reach their targets, enhancing efficacy and lowering the need for greater dosages.
Recently developed topical drug delivery systems use nanocarriers such liposomes, niosomes, solid lipid nanoparticles, and nanostructured lipid carriers. Drug encapsulation, degradation protection, and controlled release are the goals of these nanocarriers. Liposomes imitate skin lipids to improve medication penetration. Solid lipid nanoparticles provide medicine stability and controlled release appropriate for chronic skin disorders including eczema and psoriasis. Nanotechnology in topical formulations has enhanced medicine distribution and created new treatment pathways for difficult ailments. Due to their high water content, biocompatibility, and capacity to deliver hydrophilic and hydrophobic medicines, hydrogel-based systems have garnered attention in recent years. Hydrogels can heal wounds and distribute systemic drugs via transdermal patches. These cooling and relaxing devices are ideal for inflammatory skin disorders. They can also be loaded with nanoparticles or other sophisticated carriers to boost efficacy.
Bioresponsive and stimuli-responsive topical medication delivery technologies are also growing. Advanced formulations release the medicine in reaction to pH, temperature, or enzymes at the application site. For acne and infections, where the local pH is altered, pH-sensitive formulations are being investigated. Body heat releases painkillers from temperature-responsive gels. Smart delivery technologies ensure precision and reduce drug waste, advancing individualized therapy. Adding biologics like peptides, proteins, and nucleic acids to topical medication formulations is fascinating. Recent formulation advances have permitted skin administration of these complex compounds, which were previously difficult because to their size and stability. To bypass the stratum corneum and reach therapeutic levels, microneedle patches are being developed to deliver biologics gently into the skin. This technology has great potential for vaccine distribution, hormone replacement therapy, and autoimmune disease treatment.
Digital methods in topical medicine delivery are another advancement. Wearable and smart patches are being developed to monitor and manage medicine administration in real time. These systems use sensors to monitor temperature and moisture and modify medicine release. Smart dressings can monitor chronic wound healing and release antimicrobial medicines as needed, lowering infection risk and speeding recovery. Topical drug delivery and digital health technologies are changing treatment administration and monitoring. Topical medication delivery methods are important in cosmetic and dermatological industries as well as medicine. Popular goods in this segment include anti-aging creams, sunscreens, and hyperpigmentation treatments. Modern carriers like liposomes and nanoparticles have increased cosmetic formulation efficacy and stability. Consumers want eco-friendly products, therefore research into plant-based and biodegradable delivery systems is driven by demand for natural and sustainable components.
Fig. 1 Topical drug delivery system
Despite these advances, topical medication delivery system research and commercialization remain difficult. Delivering drugs consistently across skin types and circumstances is a major challenge. Topical formulations can be affected by skin permeability, which is affected by age, moisture, and disease. Patient adherence can be difficult, especially for chronic illnesses that necessitate frequent product use. Dermatologists, formulation scientists, and engineers must collaborate to solve these problems. Topical drug delivery system development also depends on regulations. FDA and EMA approval requires formulas to be safe, effective, and stable. Advanced technologies like nanocarriers and biologics require updated rules to meet strict safety criteria. For reliable evaluation of new formulations, standardized skin penetration and drug release testing methodologies are needed. The rise of personalized medicine will influence topical medication delivery technologies. Biomarkers that predict medication responses are being found thanks to genetics and proteomics. This knowledge can be utilized to create patient-specific formulations that maximize therapeutic benefits and minimize side effects. For instance, genetic insights can help create targeted treatments for psoriasis, whose causes differ by individual.
Future topical medicine delivery technologies must also address environmental sustainability. The pharmaceutical and cosmetic sectors are adopting green chemistry and producing biodegradable formulations to reduce their environmental impact. This involves drug delivery via natural polymers like chitosan and alginate. These biocompatible, eco-friendly materials support global sustainability efforts.
A rapidly growing and essential part of pharmaceutical science, topical drug delivery systems provide therapeutic chemicals directly to the skin or mucosal surfaces for localized or systemic effects. This approach is popular since it is non-invasive, easy to use, and delivers medications exactly to the target location. This discipline has advanced due to the need for improved medication bioavailability, patient compliance, and creative treatment solutions for challenging therapeutic issues. Topical drug delivery methods deposit APIs onto or within the skin to address localized diseases or achieve systemic absorption. These systems avoid the gastrointestinal tract and first-pass metabolism, decreasing systemic side effects from oral or injectable approaches. The stratum corneum, the skin's outermost layer, prevents medication penetration. Penetration enhancers, new formulation methods, and nanocarriers have been used to solve this difficulty. Today's topical formulations demonstrate this delivery method's versatility. Creams, ointments, gels, and lotions are still popular due to their simplicity and affordability. More advanced technologies including transdermal patches, liposomes, and solid lipid nanoparticles provide regulated and sustained medication release. Transdermal patches have become popular for systemic drug delivery because they release medication slowly and reduce dosage. These patches help with chronic illnesses like pain, hormone replacement, and nicotine addiction.
Nanocarriers including liposomes, niosomes, and nanostructured lipid carriers have transformed topical medication delivery. These carriers stabilize drugs, prevent API degradation, and improve skin penetration. Lipid bilayer vesicles called liposomes resemble skin lipids, enhancing medication permeability and retention. Solid lipid nanoparticles combine the benefits of classic emulsions with the stability of solid particles, making them appropriate for hydrophilic and hydrophobic medicines. These advances allow for new treatments for difficult dermatological disorders like psoriasis, atopic dermatitis, and fungal infections. Hydrogels are promising topical medication delivery vehicles. Hydrogels soothe and cool burns, wounds, and inflammatory skin conditions due to their high water content, biocompatibility, and capacity to deliver a variety of medications. Hydrogel technology has enabled hybrid systems that mix hydrogels with nanoparticles or other carriers to improve medicine delivery and efficacy. Silver nanoparticle-loaded hydrogels are useful for wound care and infection prevention due to their antibacterial properties.
Stimuli-responsive medication delivery devices are innovative topicals. Drug release is triggered by pH, temperature, or enzymes in these systems. To treat acne, pH-sensitive formulations are being created since the acidic environment can release drugs. In pain management, temperature-responsive gels release medicine when heated. These smart technologies increase drug targeting, waste reduction, and patient outcomes, advancing personalized medicine. Adding biologics to topical medication delivery devices is another innovation. Due to their bulk, fragility, and disintegration, biologics like peptides, proteins, and nucleic acids are difficult to distribute. Innovative formulation methods like microneedle encapsulation and lipid-based carriers have made skin delivery of complicated compounds possible. Microneedle patches generate skin channels that allow biologics to bypass the stratum corneum and reach therapeutic levels. This method has great potential for vaccine administration, gene therapy, and autoimmune disease treatment.
Fig. 2 Capsacin Delivery System
Digital innovations in topical medicine delivery devices have expanded this field. Wearable and smart patches are being developed to monitor and manage medicine administration in real time. These devices monitor physiological conditions including temperature, moisture, and pH to modulate medication delivery. In chronic wound management, smart dressings can monitor healing and release antimicrobial agents only when needed, eliminating overmedication and speeding recovery. Digital health and topical medicine administration are improving treatment efficiency and personalization. Cosmetic and dermatological sectors use topical medication delivery technologies. Formulation science has improved anti-aging creams, sunscreens, and hyperpigmentation treatments. Nanocarriers in cosmetics improve active ingredient penetration and stability, resulting in longer-lasting and more effective outcomes. To satisfy ecologically conscious consumers' need for natural and sustainable ingredients, plant-based and biodegradable delivery technologies have been developed.
Table 1 Topical drug delivery systems
Despite these advances, topical medication delivery system research and commercialization are difficult. Due to age, moisture, and underlying diseases, skin permeability varies greatly, making drug distribution challenging. A careful combination of science and art is needed to design medications that are effective and attractive to patients. Nanocarriers and biologics must meet strict safety and efficacy standards before being sold, which presents regulatory challenges. These issues emphasize the necessity for ongoing research and collaboration between scientists, physicians, and regulators. Topical drug delivery will evolve with customized medicine, biotechnology, and sustainability. Biomarkers that predict drug reactions are being identified by genomes and proteomics, allowing for personalized formulations that maximize efficacy and reduce side effects. Genetic knowledge of disorders like psoriasis can help design personalized medicines that target molecular pathways. In parallel, the pharmaceutical sector is applying green chemistry concepts to generate biodegradable and eco-friendly formulations. Biocompatible and sustainable carriers like chitosan and alginate are being investigated.
Literature review
Ananda et al. (2021) formulated a transdermal patch for the delivery of Primaquine (PMQ) combined with solid microneedles and a dermaroller. HPMC and glycerin were chosen as the principal polymer and plasticizer after significant optimization. Polyethylene glycol 400 (PEG 400) concentration was tuned to improve permeability. The transdermal patches were tested for weight hom ogeneity, thickness, surface pH, folding durability, moisture content, absorption, bioadhesion, and medication content recovery. Patch delivery caused no tissue injury, according to histopathology. The study showed promising results, but in vivo testing is needed to confirm them.
Mo et al. (2021) designed and tested a Carvedilol transdermal patch employing polymers and permeability enhancers to modulate drug release and maximize bioavailability without first-pass metabolism. Formulation F7, which contained Eudragit RS-100 as the rate-controlling polymer and Span 80 as a permeation enhancer, produced the best solvent-evaporated patches. Formulation F7 showed the largest drug release (100.29 ± 0.44%) within 12 hours, highest bioavailability, and maximum blood pressure reduction at six hours post-administration. A Carvedilol transdermal patch containing Eudragit RS-100 and Span 80 released medication sustainably and controlledly, according to this investigation.
Quan et al. (2021) created a transdermal patch with Siegesbeckiae Herba (SiH) extract for rheumatoid arthritis. In 2015, the UK licensed Phynova Joint and Muscle Relief Tablets™, a derivative of SiH, a traditional Chinese anti-rheumatic herbal treatment. The transdermal patch showed good anti-inflammatory and analgesic benefits, suggesting a non-invasive rheumatoid arthritis treatment.
Tahir et al. (2020) examined the recrystallization and transdermal penetration of ibuprofen and hydrocortisone in matrix-type transdermal patches loaded with polymeric and lipid nanoparticles. Polymeric nanoparticles were made from ethyl cellulose (EC4), poly(lactide-co-glycolic acid) (PLGA), and polycaprolactone (PCL), whereas MCT nanoemulsion and solid lipid nanoparticles were made from MCT and Witepsol. These nanoparticles reduced recrystallization and enabled on-demand drug loading. Controlling carrier hydrophobicity can optimize medication stability and release characteristics in transdermal patches, according to the study.
Sakdiset et al. (2019) created indomethacin (8 mg/mL) ethosomes utilizing SPC, ethanol, and additives as spreading media. With 10–30% ethanol in pH 7.4 phosphate buffer, ethosomes had higher colloidal quality. Using 4% w/v SPC:cholesterol:deoxycholic acid (6:2:1 molar ratio) in 20% v/v ethanol, the ethosomes' physical characteristics, size, and entrapment efficiency (EE) were optimized. These ethosomes penetrated indomethacin better than commercial and ethanolic solutions over 24 hours, suggesting they are better transdermal drug delivery.
Ameen and Michniak-Kohn (2019) proposed an innovative approach for drug delivery tailored primarily for Alzheimer’s disease patients, aimed at eliminating gastrointestinal side effects and improving patient compliance. They developed optimized matrix-type patches of galantamine for transdermal delivery and conducted ex vivo and in vitro evaluations. The study tested four pressure-sensitive adhesives with varying functional groups, ten diffusion enhancers, and four drug loadings to determine the optimal patch formulation.
Liu et al. (2019) developed a transdermal patch for benzoylaconitine (BA), a high molecular weight compound (603.7 Da), exhibiting strong analgesic and anti-inflammatory properties. They studied the relationship between the physicochemical properties of permeation enhancers and their enhancement efficacy using skin permeation studies. Further investigations using FT-IR and molecular modeling elucidated the enhancement mechanism, revealing that effective interaction and disruption of both lipophilic and hydrophilic domains of the stratum corneum were critical for achieving significant permeation improvement.
Zhou et al. (2018) explored transdermal drug delivery systems for local and systemic drug administration. They highlighted that conventional physical and chemical enhancer, while effective at improving drug solubility and diffusion, often cause toxicity due to excessive enhancer penetration. Nano-formulations, ranging from 10 to 100 nm in size, were suggested as a promising alternative. Their smaller size enhanced treatment permeability, stability, and targeting. Applications of nano-formulations, such as vesicles, nanoparticles, and nanoemulsions, were extensively studied for transdermal drug delivery.
Tosato et al. (2018) developed a transdermal drug delivery system for trans-resveratrol (RSV), a natural compound with anti-inflammatory and anticancer activity. Using liposomal nanoparticulate carriers, including conventional, deformable, and ultradeformable liposomes, they performed detailed characterization with techniques like transmission electron microscopy, dynamic light scattering, and confocal Raman spectroscopy. The formulations enhanced bilayer fluidity, improved drug encapsulation, and maintained carrier mobility, offering potential for efficient transdermal delivery.
Kathe and Kathpalia (2017) emphasized the importance of topical drug delivery systems, which rely on the drug's physicochemical properties and the adherence of formulations to the skin. They addressed limitations of traditional systems, such as poor skin adhesion and permeability, by developing topical film formulations. These systems formed thin, transparent films that adhered to the skin and provided sustained drug release, enhancing therapeutic efficacy and patient satisfaction.
Siji et al. (2016) investigated the effect of backing films on the transdermal delivery of cyclobenzaprine patches. Various backing films were evaluated for drug release rates using in vitro studies. ATR-FTIR spectroscopy revealed strong adsorption of cyclobenzaprine onto certain backing films, such as Cotran™ 9700, which affected drug release after storage. Their findings highlighted the importance of material compatibility in transdermal patch design.
Indulekha et al. (2016) developed a temperature-responsive transdermal drug delivery system (TDDS) using a thermoresponsive polymer, poly(N-vinyl caprolactam) (PNVCL). The system allowed controlled drug release via heat application. By incorporating PNVCL into a pH-sensitive chitosan biopolymer, the team created a co-polymer gel responsive to both temperature and pH. Drug release studies demonstrated enhanced permeation at elevated temperatures, and biocompatibility testing confirmed the system's safety.
Gundeti et al. (2015) formulated transdermal patches for Nateglinide using a solvent casting method and various polymer combinations. The patches were characterized for homogeneity, drug content, and permeation properties. The optimized formulation achieved sustained drug release for 12 hours (99.2%) and maintained stability per ICH guidelines.
Zhang et al. (2014) optimized a transdermal patch for Diclofenac using organic amine salts as permeation enhancers. Using excised rabbit skin in diffusion cells, they determined that Diclofenac triethylamine salt exhibited superior penetration compared to other salts, demonstrating the importance of enhancer selection for effective transdermal delivery.
Laxmi et al. (2014) prepared bilayer films for wound healing, combining a Diclofenac-loaded upper layer and a drug-free lower layer for controlled release. In vitro studies showed sustained drug delivery, highlighting the potential of bilayer films for prolonged therapeutic effects.
Panchaxari et al. (2013) evaluated transdermal patches of Diclofenac diethylamine using silicone and acrylic adhesives. They concluded that an optimal adhesive combination provided sustained drug release, improved permeation, and robustness.
Bhavya et al. (2012) developed a potassium Diclofenac transdermal system using polyvinylpyrrolidone and guar gum polymers. Kinetic studies indicated sustained drug release following a non-Fickian model, validating the system's sustained action potential.
Shingade et al. (2012) reviewed transdermal drug delivery methods, emphasizing their ability to bypass hepatic first-pass metabolism and maintain steady plasma drug levels, improving therapeutic efficiency.
Subramanian et al. (2012) formulated a matrix patch for Fexofenadine Hydrochloride with controlled release properties. In vitro studies showed 94% drug release in 23 hours, while in vivo studies confirmed 99% release in 24 hours, following zero-order kinetics.
Lion and Brennan (2010) reviewed the clinical effectiveness of Diclofenac epolamine topical patches for treating soft tissue injuries. They noted the advantages of lower plasma drug concentrations and fewer systemic side effects compared to oral NSAIDs.
Kevin et al. (2009) developed a monolithic matrix transdermal formulation of Tramadol HCl and demonstrated sustained release through in vitro studies using Franz diffusion cells.
Chandrashekar and Sobha (2008) discussed the criteria for selecting drugs suitable for transdermal delivery, focusing on pharmacokinetic and physicochemical parameters.
Capsaicin, the main ingredient in chili peppers, is of interest to pharmaceutical and biomedical researchers. Effective analgesic and anti-inflammatory qualities make capsaicin essential in pain management formulations. New extraction technologies and transdermal drug delivery systems can improve bioavailability, therapeutic effects, and systemic side effects. This section review examines capsaicin extraction, transdermal patch composition, and evaluation. Capsaicin purity and efficacy for pharmaceutical uses depend on chili pepper extraction. Soxhlet extraction and maceration use organic solvents such ethanol, methanol, and acetone. These procedures are time-consuming and may degrade thermolabile components. Modern methods are more efficient and eco-friendlier. Supercritical fluid extraction (SFE) uses carbon dioxide as a solvent to produce pure capsaicin with high yields and low environmental effect. This approach is useful since it preserves the compound's bioactivity at lower temperatures.
Another new method, ultrasound-assisted extraction (UAE), disrupts plant cell walls to liberate capsaicin. UAE improves extraction efficiency, processing time, and solvent use, according to studies. Microwave-assisted extraction (MAE) is used for uniformly heating the extraction medium, maximizing capsaicin production. Pharmaceutical research is emphasizing green chemistry and sustainable practices, which these improved methods support. Transdermal Patch Formulation
Transdermal drug delivery systems (TDDS) are promising alternatives to conventional medication delivery. These systems bypass the gut, lowering first-pass metabolism and increasing bioavailability. Transdermal capsaicin patches provide capsaicin locally to the pain site, reducing systemic exposure and side effects. Capsaicin is added to a polymeric matrix, the patch's drug reservoir. Biocompatibility and mechanical strength make HPMC, ethyl cellulose, and PVA popular. The patch's adhesiveness, medication release, and efficacy depend on the polymer. Recent studies have examined how nanotechnology can improve capsaicin transdermal patches. Drug dispersion and controlled release are improved by adding nanoparticles and nanofibers to the polymeric matrix. Capsaicin's poor water solubility can be improved by adding lipid-based nanoparticles. Adding permeation enhancers like menthol and ethanol helps capsaicin penetrate the stratum corneum, the outermost layer of the skin.
Transdermal patches are evaluated for physicochemical qualities, drug release kinetics, and therapeutic efficacy. Patch thickness, tensile strength, wetness, and medication loading efficiency matter. Drug delivery and patient compliance are congruent with these qualities. In vitro investigations are essential for understanding capsaicin patch medication release. Franz diffusion cells are used to test capsaicin penetration through synthetic membranes or animal skin. These experiments illuminate the patch's zero-order kinetics release profile for sustained medication administration. Bioadhesive polymers also increase the patch's skin residence period, boosting its therapeutic potential. Clinical trials are essential for capsaicin transdermal patch efficacy and safety. They effectively treat neuropathic pain, osteoarthritis, and postherpetic neuralgia, according to recent studies. A capsaicin 8% patch randomized controlled trial showed considerable pain reduction in diabetic neuropathy patients. These experiments further emphasise the need of patch design optimization to reduce skin irritation and burning.
Despite hopeful advances, capsaicin transdermal patch composition and evaluation remain difficult. Capsaicin's pungency and skin irritation require cautious excipient and permeation enhancer selection. Scalability of modern extraction and patch manufacturing methods is another issue for commercial production. Researchers are investigating microencapsulation and co-crystallization to solve these problems. Capsaicin is microencapsulated in a biocompatible shell to control release and reduce discomfort. However, co-crystallization with proper co-formers improves capsaicin solubility and stability. Hydrogel-based technologies and bio-responsive polymers could lead to next-generation capsaicin patches. High water content and flexibility make hydrogels ideal for long-term usage due to their comfort and adherence. Bio-responsive polymers that release medications in reaction to temperature or pH could improve pain management. Additionally, patch design and optimization are increasingly using AI and ML. These methods forecast medication release profiles, optimize formulation parameters, and speed development. Research discoveries should be translated into clinically viable products faster using such advancements.
Table 2 Review on extraction of capsaicin: formulation and evaluation of a transdermal patch
Due to its analgesic, anti-inflammatory, and anti-obesity characteristics, capsaicin, a bioactive chemical found in chili peppers of the family Capsicum, is an important molecule in pharmacological research. It works by attaching to TRPV1 receptors to desensitize pain and heat. Its medicinal potential is well known, but poor water solubility, skin permeability, and irritation require new administration techniques such transdermal patches. Capsaicin-based transdermal patches bypass the gastrointestinal system and deliver this ingredient sustainably at the application site. Extracting capsaicin from natural sources is crucial to medicinal use. Chili peppers' dried fruit contains capsaicin, which varies by species, maturity, and climate. Soxhlet extraction and maceration use organic solvents such ethanol, methanol, or acetone. Recently developed green chemistry methods like supercritical fluid extraction (SFE) employing carbon dioxide preserve capsaicin's bioactivity without solvents. Solvent polarity, extraction temperature, and duration are tuned for optimal yield and purity, which are essential for product efficacy.
Capsaicin must be characterized to ensure purity and chemical composition after extraction. HPLC, GC-MS, and FTIR are commonly used. These methods check capsaicin purity and discover related chemicals like dihydrocapsaicin, which may affect its pharmacological characteristics. Pharmaceutical formulation repeatability and scalability require extraction and analytical methodology standardization. Capsaicin's hydrophobicity and low molecular weight must be addressed while making a transdermal patch. Transdermal patches administer capsaicin to systemic circulation or specific regions. To increase skin permeability, ethanol, propylene glycol, or natural terpenes must be added. The patch matrix, made of HPMC, ethyl cellulose, or polyvinyl alcohol, provides structural stability and controls capsaicin release.
Excipients and patch manufacture affect capsaicin patch medication release kinetics. Solvent casting, where drugs and polymers are dissolved in a solvent and cast onto a backing membrane, is common. Advanced fabrication methods including electrospinning and 3D printing improve patch matrix medication distribution precision. To improve stability, bioavailability, and skin irritation, capsaicin has been encapsulated in lipid nanoparticles or nanospheres. Capsaicin transdermal patch performance evaluation requires in vitro, ex vivo, and in vivo research. Franz diffusion cells assess drug penetration via synthetic membranes or excised animal skin in vitro. These investigations reveal release profile, permeation rate, and penetration enhancer effects. Ex vivo investigations on human or animal skin recreate patch-biological tissue interaction more realistically. To ensure patient compliance and comfort, adhesive strength, patch flexibility, and water vapor transmission rate are assessed. Capsaicin patch efficacy and safety must be assessed in vivo. Capsaicin pharmacokinetics and pharmacodynamics are studied in animals. Optimizing the formulation involves measuring Tmax, half-life, and bioavailability. Phased human clinical trials evaluate the patch's safety, efficacy, and side effects in specific populations. Due of capsaicin's skin irritation and burning effects, dose optimization and soothing medications are needed.
Capsaicin transdermal patches can treat osteoarthritis, postherpetic neuralgia, and sports medicine irritants. Locally acting capsaicin lowers systemic adverse effects, making it safer than oral or injectable analgesics. Transdermal patches also prolong therapeutic benefits, eliminating the need for frequent administration. Capsaicin and other medications, such as NSAIDs, are being studied in a patch for pain control synergy. Despite these benefits, capsaicin transdermal patch development is difficult. Skin irritation is a major issue, especially in sensitive people. Reducing capsaicin concentration, adding anti-inflammatory drugs, or inventing controlled-release encapsulation technologies can help. Another issue is skin permeability, which varies by age, ethnicity, and skin condition. These concerns are being addressed by personalizing patch compositions to patient demands.
Capsaicin patches need regulatory approval to be sold. FDA and EMA quality control standards must be met by the patch. Displaying medication content consistency, release uniformity, and stability under various storage circumstances. Since adverse events and user input inform future product developments, post-marketing surveillance ensures long-term product safety and efficacy. With continuous research enhancing formulation and delivery, capsaicin-based transdermal patches have a bright future. Biomaterials like bioadhesive polymers and stimuli-responsive hydrogels can improve capsaicin patch performance. Wearable technology like patches with sensors that monitor drug release or skin problems are fresh approaches to personalized treatment. Combining capsaicin with new drug delivery methods like microneedles or iontophoresis may overcome transdermal delivery's limitations.
Chili peppers contain capsaicin, an alkaloid with analgesic, anti-inflammatory, and anti-obesity characteristics that have garnered attention in the pharmaceutical and biomedical industries. Chili peppers' pungency comes from this bioactive molecule, which has been researched for its pain-relieving properties. Capsaicin works by attaching to sensory neuron TRPV1 receptors. These receptors desensitize pain and heat, making capsaicin an excellent treatment for neuropathic pain, osteoarthritis, and postherpetic neuralgia. However, capsaicin oral administration can cause gastrointestinal irritation and low absorption, requiring other delivery routes. Controlled release and patient compliance make transdermal patches a viable option. This topic covers capsaicin extraction, transdermal patch formulation, and complete evaluation.
Capsaicin extraction is essential for pharmaceutical applications. The most common chili peppers used to make capsaicin are Capsicum annuum and Capsicum frutescens. Capsaicin concentration in chili peppers depends on species, cultivation, and harvest maturity. Maceration, Soxhlet, and supercritical fluid extraction have been used to extract pure capsaicin. Maceration and Soxhlet extraction dissolve capsaicin from dried chili powder using organic solvents such ethanol, methanol, or acetone. These technologies work, but they require substantial solvent recovery and purification, making them less environmentally friendly. However, supercritical fluid extraction, especially utilizing carbon dioxide, has become popular due to its eco-friendliness and ability to preserve capsaicin bioactivity. High pressures and moderate temperatures allow SFE to selectively extract capsaicin without thermal degradation. To maximize yields and purity, pressure, temperature, and co-solvent type (ethanol) are tuned. This method improves extraction efficiency and follows green chemistry, making it suited for large-scale production. No matter the method, post-extraction purification with chromatography removes contaminants and related chemicals like dihydrocapsaicin, which might affect the product's pharmacological effects.
Characterizing extracted capsaicin is essential for pharmacological use. HPLC, GC-MS, and FTIR are used to determine capsaicin purity, content, and structural integrity. These procedures give researchers complete analytical profiles to standardize the chemical for formulation. Maintaining therapeutic efficacy and reducing impurity adverse effects requires high purity. The formulation of capsaicin into a transdermal patch requires materials science, pharmacology, and drug delivery engineering. Drugs are delivered using transdermal patches, bypassing the gastrointestinal route and liver first-pass metabolism. Capsaicin's hydrophobicity and low molecular weight hinder skin penetration. The patch recipe includes penetration boosters such ethanol, propylene glycol, or natural terpenes to solve these difficulties. These enhancers disturb the stratum corneum, the outermost skin layer, allowing capsaicin to penetrate deeper.
Another important formulation design concern is patch matrix polymer selection. Due to its biocompatibility, flexibility, and drug release control, HPMC, ethyl cellulose, and polyvinyl alcohol are widely employed. The patch matrix must be strong enough to stick to the skin and remove painlessly. Transdermal patches are often made via solvent casting. After dissolving the medication and polymers in a solvent and casting them onto a backing layer, they dry to produce a homogeneous matrix. New methods like electrospinning and 3D printing provide precise medication distribution and patch shape, improving system performance.
New nanotechnology has enhanced capsaicin transdermal patches. Encapsulating capsaicin in lipid nanoparticles, liposomes, or polymeric nanospheres improves stability, bioavailability, and skin permeability. These nano-carriers restrict capsaicin release, minimizing skin irritation from high concentrations. Biocompatibility and adverse reactions can be improved by adding natural polymers like chitosan or alginate to the patch matrix. Capsaicin transdermal patches are tested in vitro, ex vivo, and in vivo for safety, effectiveness, and performance. Franz diffusion cells assess drug penetration via synthetic membranes or excised animal skin in vitro. These investigations reveal capsaicin release kinetics, penetration enhancer effects, and patch medication delivery effectiveness. Ex vivo experiments on human or animal skin imitate the patch's interaction with biological tissues, revealing its irritation, adherence, and flexibility.
In vivo studies are needed to assess capsaicin patch pharmacokinetics and pharmacodynamics in animals and humans. Optimizing the formulation involves analyzing Tmax, elimination half-life, and bioavailability. Phased human clinical trials evaluate the patch's safety and efficacy in selected populations. To reduce patient discomfort, capsaicin's well-known adverse effects—burning and skin irritation—require dose adjustment and soothing medicines like aloe vera or menthol. Therapeutic uses of capsaicin transdermal patches go beyond pain treatment. These patches may help treat diabetic neuropathy, rheumatoid arthritis, and weight loss by modulating energy metabolism. Localized capsaicin patches lessen systemic adverse effects, making them safer than oral or injectable analgesics. Transdermal patches' continuous release prolongs therapeutic benefits, reducing administration frequency and boosting patient adherence.
Fig. 3 Developing Capsaicin Trans-dermal Patch
For widespread usage, capsaicin transdermal patches must overcome many obstacles. Skin irritation is a major problem, especially for sensitive skin or dermatological diseases. Reducing capsaicin levels, adding anti-inflammatory medications, or encapsulating drugs can help. Another issue is skin permeability, which varies by age, ethnicity, and skin wetness. Personalized patch design, including patches for individual skin types or disorders, is being investigated to address these variances. Regulatory approval is crucial for capsaicin transdermal patch development. The FDA and EMA require these items to meet strict quality criteria. Displaying medication content consistency, release uniformity, and stability under various storage circumstances. Post-marketing surveillance guarantees product safety and efficacy, and user input informs future developments. Capsaicin transdermal patches will benefit from new materials and technologies. Stimuli-responsive hydrogels which release drugs when temperature or pH changes provide regulated and targeted administration. A unique approach to personalized medicine uses wearable sensors in patches to monitor drug release or skin problems in real time. In a patch, capsaicin and other medicinal medicines like NSAIDs may work synergistically to treat complex disorders like rheumatoid arthritis.
References
Ameen D and Michniak-Kohn B; 2019, Development and in vitro evaluation of pressure sensitive adhesive patch for the transdermal delivery of galantamine: effect of penetration enhancers and crystallization inhibition, European Journal of Pharmaceutics and Biopharmaceutics..
Ananda PWR, Elim D, HilmanSyamami Zaman, WahdaniyahMuslimin, Muhamad Gilang Ramadhan Tunggeng, Andi Dian Permana, Combination of transdermal patches and solid microneedles for improved transdermal delivery of primaquine, International Journal of Pharmaceutics, 609, 2021, 121204,
Arunachalam A; Karthikeyan M; Kumar VD; Prathap M; Sethuraman S; Ashutoshkumar S; Manidipa S; 2010, Transdermal drug delivery system: a review; Current Pharma Research; 1, 1, 70- 81.
Berasain C., Castillo J., Perugorria M. J., Latasa M. U., J. Prieto, and M. A. Avila, “Inflammation and liver cancer: new molecular links,” Annals of the New York Academy of Sciences, vol. 1155, pp. 206–221, 2009.
Bhavya B B; Shivakumar H R; Vishwanath B; 2012, in- vitro drug release behavior of pvp/guar gum polymer blend transdermal film with diclofenac potassium; Masian Journal Of Pharmaceutical And Clinical Research; 5, 2, 149152.
Calabro P., Chang D. W, Willerson J. T., Yeh E. T. H., “Release of C-reactive protein in response to inflammatory cytokines by human adipocytes: linking obesity to vascular inflammation,” Journal of the American College of Cardiology, vol. 46, no. 6, pp. 1112– 1113, 2005.
Chandrashekar NS; Shobha RH; 2008, Physicochemical and pharmacokinetic parameters in drug selection and loading for transdermal drug delivery, Indian Journal of Pharmaceutical Sciences; 94-96.
Characterization Of Monolithic Matrix Transdermal Systems Using HPMC/Eudragit S 100 Polymer Blends,International Journal Of Pharmacy And Pharmaceutical Sciences, 1, 1, 108-120.
Cherukuri S, U. R. Batchu, K. Mandava, V. Cherukuri, K. R. Ganapuram, 2017, Formulation and evaluation of transdermal drug delivery of topiramate, Int. J. Pharm. Investig, 7,10–17.
Chobanian AV, Bakris GL, Black HR, Cushman WC, Green LA, Izzo JL, Jones DW, Materson BJ, Oparil S, Wright JT, Roccella EJ, 2003. The Seventh Report of the Joint National Committee on Prevention, Detection, Evaluation, and Treatment of High Blood Pressure: The JNC 7 Report. JAMA; 21, 289(19), 2560-72.
Chojnicka A, Sala G, Cornelus G, 2009, The interaction between oil droplets and gel matrix affect the lubrication properties of sheared emulsion filled gels, Food hydrocolloids, 23, 1038‐1046.
Coussens L. M. and Werb Z., “Inflammation and cancer,” Nature, vol. 420, no. 6917, pp. 860–867, 2002.
Cynthia LS; John TS; Robert L; 2012, Reservoir-based drug delivery systems utilizing microtechnology; Advanced Drug Delivery Reviews; 64, 14, 1590– 1602.
Eaves-Pyles T.,. Allen C. A, Taormina J.et al., “Escherichia coli isolated from a Crohn's disease patient adheres, invades, and induces inflammatory responses in polarized intestinal epithelial cells,” International Journal of Medical Microbiology, vol. 298, no. 56, pp. 397–409, 2008.
Fathima SA; Begum S, Fatima SS, 2017, Transdermal drug delivery system; International Journal of Pharmaceutical and Clinical Research; 9, 1, 35-43.
Ferguson L. R, “Chronic inflammation and mutagenesis,” Mutation Research— Fundamental and Molecular Mechanisms of Mutagenesis, vol. 690, no. 1-2, pp. 3– 11, 2010.
Gabay C, Kushner I (1999) Acute-phase proteins and other systemic responses to inflam- mation. New Engl J Med 340:448–455.
Gowramma B; Babu B; Krishnaveni N; Meyyanathan SN; Elango K; Suresh B; 2012, A RP-HPLC method for simultaneous estimation of aceclofenac, paracetamol and tizanidine hydrochloride in pharmaceutical formulation; Journal of Pharmacy Research; 5, 3, 1313-1315.
Gundeti A; Aparna C; Srinivas P; 2015, Formulation and evaluation of transdermal patch and gel of nateglinide human, International Journal of Pharmacy and Pharmaceutical Research; 4, 2, 339-357.
Hadgraft J; Skin: The final frontier; International Journal of Pharmaceutics; 224, 1-8 (2001).
Indulekha S; Arunkumar P; Bahadur D; Srivastava R; 2016,
Kaplan NM, 2002. Kaplan’s clinical hypertension. 8th ed. Philadelphia, Lippincott Williams and Wilkins.
Kathe K and Kathpalia H; 2017, Film forming systems for topical and transdermal drug deliver;, Asian Journal of Pharmaceutical Sciences, 12, 6, 487-497.
KelebE,SharmaRk, Mosa EB, 2010, Transdermal drug delivery systemdesign and evaluation. International journal of advances in pharmaceutical science, I, 201-211.
Kevin CG, Shinde AJ, Shah PH, 2009, Formulation And In-Vitro
Kreilgaard M, 2001, Dermal pharmacokinetics of microemulsion formulations determined by in vivo microdialysis, Pharmaceutical Research, 18, 3, 367-373. Kshirsagar NA, 2000, Drug Delivery Systems, Ind. J. Pharmacol, 32, S54‐S61.
Laxmi P, Mohammad A, Shirisha G, 2014, Development and Characterization of Bilayered Transdermal Film of Diclofenac Diethylamine., IJPSR, 5, 6, 2518-2524.
Lembhe S, Dev A, 2016, Transdermal drug delivery system: An overview. World J Pharm PharmSci, 5:584-610.
Lim SS, Vos T, Flaxman A, Danaei G, Shibuya K, Rohani HA, 2012. A comparative risk assessment of burden of disease and injury attributable to 67 risk factors and risk factor clusters in 21 region, 1990–2010: a systematic analysis for the Global Burden of Disease Study 2010. The Lancet; 380 (9859), 2224-2260.
Lion DR and Brennan BMJ, 2010, Topical Nonsteroidal Anti-Inflammatory Drugs For The Treatment Of Pain Due To Soft Tissue Injury: Diclofenac Epolamine Topical Patch Journal Of Pain Research, 3 223–233.
Liu C; Farah N; Weng W; 2019, Investigation of the permeation enhancer strategy on benzoylaconitine transdermal patch: The relationship between transdermal enhancement strength and physicochemical properties of permeation enhancer,
Markiewski M. M. and Lambris J. D., “The role of complement in inflammatory diseases from behind the scenes into the spotlight,” American Journal of Pathology, vol. 171, no. 3, pp. 715–727, 2007
Mo L, Guijing Lu, XipingOu, Dongsheng Ouyang, Formulation and development of novel control release transdermal patches of carvedilol to improve bioavailability for the treatment of heart failure, Saudi Journal of Biological Sciences, 2021, ISSN 1319-562X,
Narajji C, Patel HR, Karvekar MD, Babu S, 2011, Simultaneous Estimation of Aceclofenac, Paracetamol and Tizanidine in Their Combined Dosage Forms by Spectrophotometric and RP- HPLC Method, Journal of Analytical & Bioanalytical Techniques :DOI: 10.4172/2155-9872.
Nathalie F, Valery G, Marie JS, 2004, The release of caffeine from hydrogenated and fluorinated gel emulsion and cubic phases, Collo. and Surf. A, 243, 117‐125.
Nicklas B. J., Ambrosius W., Messier S. P. et al., “Diet-induced weight loss, exercise, and chronic inflammation in older, obese adults: a randomized controlled clinical trial,” The American Journal of Clinical Nutrition, vol. 79, no. 4, pp. 544–551, 2004.
Panchaxari DM, Pampana S, Pal T, Devabhaktuni B, Aravapalli AK, 2013, Design And Characterization Of Diclofenacdiethylamine Transdermal Patch Using Silicone and Acrylic Adhesives Combination. Daru Journal of Pharmaceutical Sciences, 21, 6, 1-14.
Pecchi, M. Dallaporta, A. Jean, S. Thirion, and J.-D. Troadec, “Prostaglandins and sickness behavior: old story, new insights,” Physiology and Behavior, vol. 97, no. 34, pp. 279– 292, 2009.
Phanindra S and Sujani PV, 2010, Validated HPTLC Method for the Simultaneous Determination of Diclofenac Potassium and Tizanidine Hydrochloride in Tablet Dosage Form Research Journal of Pharmaceutical, Biological and Chemical Sciences, 1, 1.
Prasada RK and Sharma R, 2010, Simultaneous estimation and validation of Rabeprazole Sodium and Diclofenac Sodium in capsule dosage form: J. Chem. Pharm. Res., 2(2): 186-196: ISSN No: 0975-7384.
Prausnitz M.R., 2004, Microneedles for transdermal drug delivery. Adv Drug Deliv Rev 56: 581–587.
Quan P, Jiao B, Shang R, Liu C, Liang Fang, Alternative therapy of rheumatoid arthritis with a novel transdermal patch containing SiegesbeckiaeHerba extract, Journal of Ethnopharmacology, 265, 2021, 113294, ISSN 0378-8741.
Rieger MM, Lachman L, Lieberman Ha, KanigJl, 1986, Emulsions. The Theory and Practice of Industrial Pharmacy. 3rd ed. Philadelphia, PA: Lea and Febiger; p 502‐533.
Roderick B. Walker, Eric W. Smith, 1996, The role of percutaneous penetration enhancers, Advanced Drug Delivery Reviews 18; 295-301.
Rowe RC, Sheskey PJ, Quinn ME. In Handbook of Pharmaceutical Excipients. 6th Edn; Philippines: PhPAPhA Pharmaceutical Press 2009, pp 298-300, 478480,782-784.
Sakdiset P; Amnuaikit T; Pichayakorn W; Pinsuwan S; 2019, Formulation development of ethosomes containing indomethacin for transdermal delivery; Journal of Drug Delivery Science and Technology; 52, 760-768.
Schmid-Schönbein G. W., “Analysis of inflammation,” Annual Review of Biomedical Engineering, vol. 8, pp. 93–151, 2006.
Sheikh S, Faiyaz S, Talegaonkar S, Ali J, Baboota S, Alka A and Khar RK, 2007, Formulation development and optimization using nanoemulsion technique: A technical note, Ame Pharm Sci. Pharm Sci Tech, 8, E1‐E6.
Shingade GM, Aamerquazi, Sabale P, Grampurohit N, Gadhave M, Jadhav S, Gaikwad D. 2012, Review On: Recent Trend On Transdermal Drug Delivery System Journal Of Drug Delivery & Therapeutics; 2 (1) 66.
Siji L; Peng Q; Liu X; Fang L; 2016, Effect of backing films on the transdermal delivery of cyclobenzaprine patch asian journal of pharmaceutical Sciences; 11; 780–783.
Subramanian S, Raj Kapoor B, Vijayaraghavan C, 2012, In-Vitro And In-Vivo Study Of Fexofenadine Hydrochloride Transdermal Patches, International Journal Of Pharmaceutical And Chemical Sciences, 1,1, 343-346.
Tahir MA, Ali ME, Alf Lamprecht, Nanoparticle formulations as recrystallization inhibitors in transdermal patches, International Journal of Pharmaceutics, 575, 2020, 118886,
Thermoresponsive polymeric gel as an on-demand transdermal drug delivery system for pain management, Materials Science and Engineering: C, 62, 113122.
Tosato MG; Maya JV; Martin AA; Tippavajhala V; Mele M; Dicelio L; 2018, Comparative study of transdermal drug delivery systems of resveratrol: High efficiency of deformable liposomes, Materials Science and Engineering, 90, 356364.
Zhang Y; Cun D; Kong X; Fang L; 2014, Design and evaluation of a novel transdermal patch containing diclofenac and teriflunomide for rheumatoid arthritis therapy; Asian journal of pharmaceutical sciences; 9; 251-259.
Zhou X; Hao Y; Yuan L; Pradhan S; Shrestha K; Pradhan O; Li W; 2018, Nanoformulations for transdermal drug delivery: a review; Chinese Chemical Letters; doi:10.1016/j.cclet.2018.10.037.
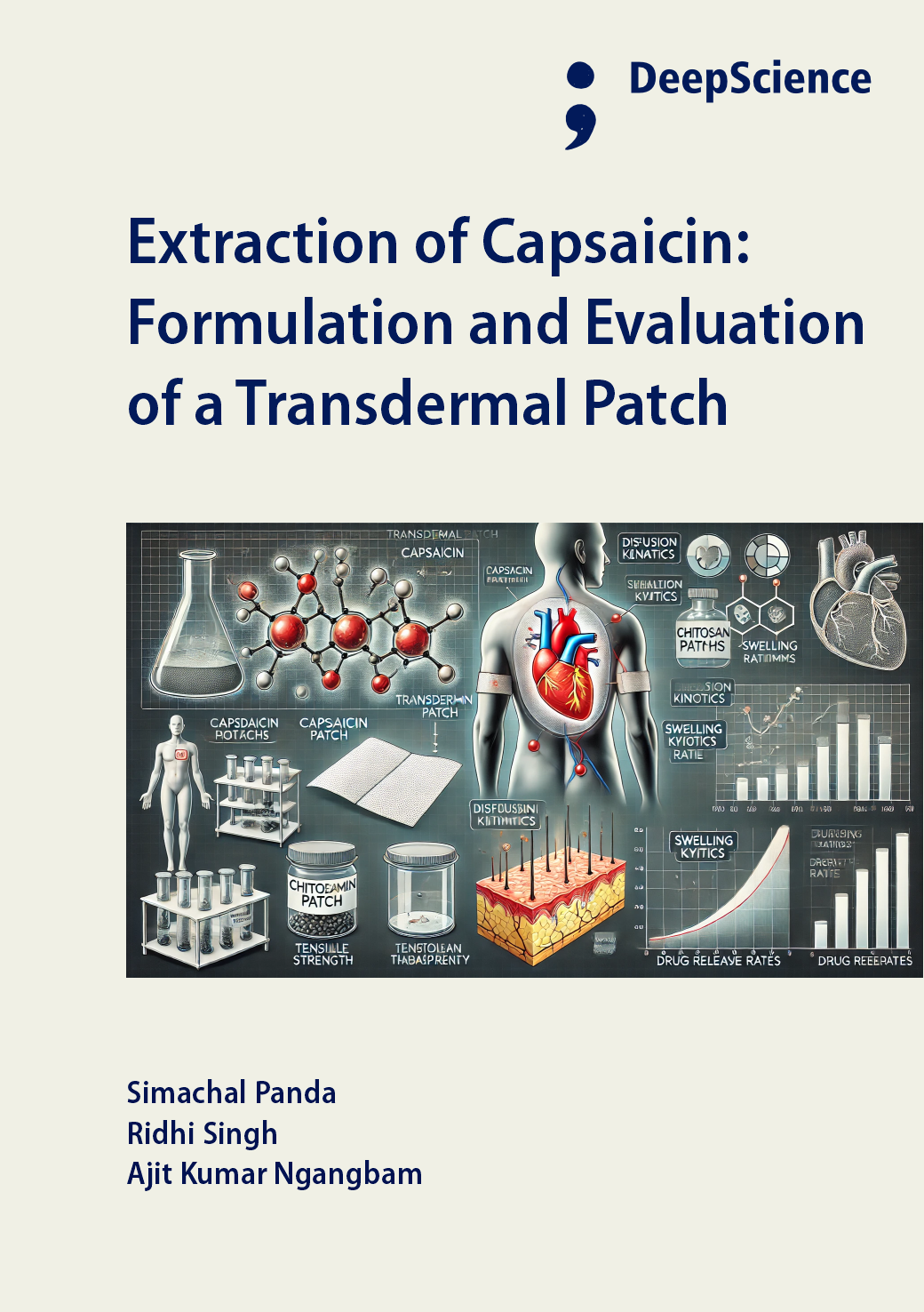